In previous issues of the radar blog we have reviewed the main characteristics of MMIC transceivers for automotive radar. Yet, an aspect still needs to be covered: how to integrate the MMIC on the radar module’s PCB, and what should we consider to optimize the interface between the chip and the antennas. For that, we need first to consider what kind of antenna is used in the radar system. As an example, we will consider here two of the most widely spread solutions: microstrip patch antenna arrays and slotted waveguides.
In FMCW radar systems, the antennas are used to transmit a continuous electromagnetic wave, modulated in frequency over time. The signal reflected back from objects in the environment is also received by the antennas and fed it back into the system for processing. Broadband Horn Antenna
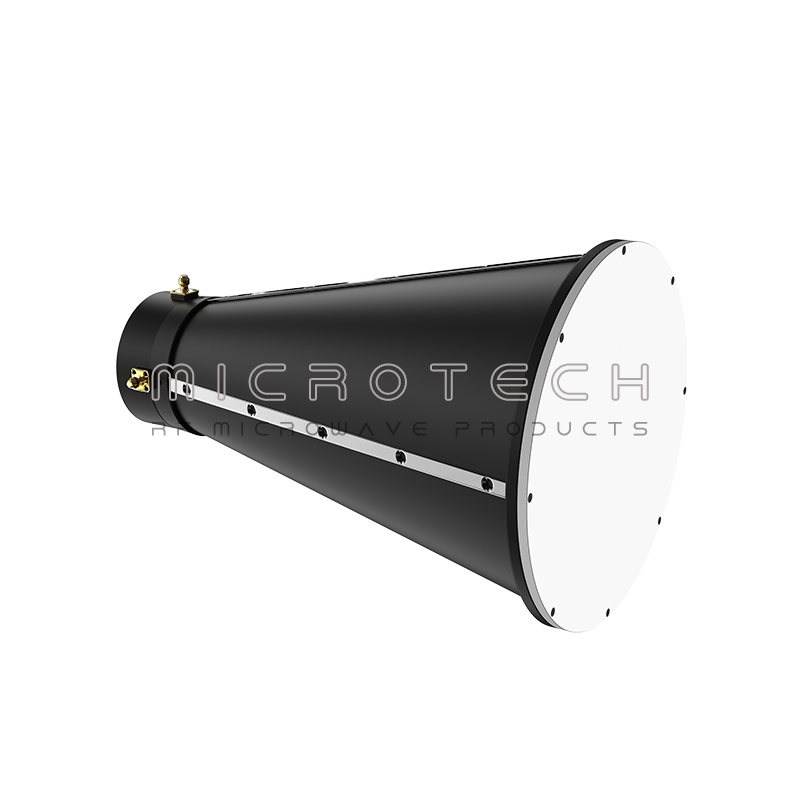
When designing antennas for FMCW automotive radar modules, there are some important aspects, which need to be considered. First, the antenna bandwidth should be sufficient to cover the frequency band of interest (76-77GHz for long range radar, 77-81GHz for corner and imaging radar, depending on the region and standards) while keeping a good matching and radiation properties. The antenna gain and beamwidth must be ensured over all the frequency sweep range, to warrantee the desired range and field of view.
As mentioned in previous blog entries, antennas arrays with half-wavelength separation between the rows are frequently used, to maximize the field of view. Yet this can lead to strong mutual coupling between the antenna elements, leading to degraded performance. The antenna should also be designed to minimize any interference or reflections from other components on the board, the module casing and vehicle parts such as fascia or emblems.
Finally, the antenna also has to be as small and compact as possible to fit within the available space allocated to the radar module without losing efficiency, while keeping the manufacturing and assembly costs as low as possible. The easiest option would be to use planar antennas directly printed on the PCB board, but the technological advances in manufacturing have fostered the interest in 3D antennas, which include waveguide-based geometries and lenses.
For many years, microstrip patch antennas have been the most popular radiators for automotive radar systems, due to their compact size, low profile, and directional radiation pattern. They were also able to cover the frequency band for radar applications with low to moderate bandwidth. In addition, they are easy to integrate, as they are directly printed on the PCB, and do not require additional components or manufacturing steps.
Patch antennas consist of metallic structures printed on the PCB, over a ground plane. The most common antennas are series-fed linear patch arrays, yet other structures like comb-line antennas or corporate-fed patch arrays can also be found.
In a linear patch array, the length of the individual patches will determine the frequency of operation, while changing the patch width will determine the amplitude contribution of the element to the total radiation. The phase distribution is set by the spacing between the elements in the array and the length of the line that connects them. Therefore, by adjusting the widths and spacing, the radiation patterns can be shaped to match the required properties, such as low sidelobe levels to reduce interference.
The high frequency of operation of mmWave radar creates challenges for the design of patch antenna arrays. The losses in the feeding lines to the antennas can be significant, which translates into a loss of efficiency and gain of the patch arrays. The losses are to the dielectric material of the PCB and to the high resistance of the metallic lines. It is therefore a good practice to keep the lines as short as possible, while using high quality laminates as a substrate for the layer where the antennas are printed.
Additionally, the bandwidth of series-fed patch arrays is limited by the bandwidth of the individual patch elements on the thin material used for the PCB. Additionally, the feeding lines can further limit the achievable bandwidth. Also, it is necessary to control exactly the phase of the signal that is fed to each element, to achieve a constructive addition of the radiation in the desired direction. This is set by the length of the line between the patches, and the distance between them. The effect is frequency dependent, which will lead to a certain variation of the gain and pointing direction along the frequency band of operation.
We have discussed in previous entries that the optimum distance between antennas for maximum field of view is half a wavelength. This means that the adjacent patches in a serial fed patch antenna array are closely spaced, which can result in high mutual coupling, which can degrade the performance of the array. Also, in some cases high cross-polarization levels can appear at some frequencies, reducing the overall performance of the antenna.
All these aspects make the design of patch antennas for automotive radar a delicate task, which requires the use of advanced electromagnetic simulation tools and extensive skills and experience.
Manufacturing tolerances will also have a strong influence on the performance of patch array antennas in the automotive radar bands. Etching tolerances, defective surface finish and variations in the material parameters will have a significant effect on the performance. To keep the required consistency, high quality materials and high precision manufacturing fabrication techniques are required, which increases the cost of the boards, and therefore of the whole system.
Slotted waveguide antennas are also widely used in automotive radar systems due to their good performance, high reliability, and ease of integration. One of their key advantages over other antenna types is that they can operate at higher frequencies with lower losses, as the propagation medium in the waveguide is air, which virtually eliminates all dielectric losses. The waveguide is typically made of a conductive material. In the past, copper or aluminum would be used, with the slots etched using high precision machining. But with the advances in manufacturing technologies, metalized plastic injection molding and 3D printing technologies are becoming more and more popular.
Slotted waveguide antennas consist of one or more rectangular waveguides with narrow slots cut into the wider walls. These slots act as radiators, fed with the signal transmitted through the waveguide. The size, shape and position of the individual slots on the waveguide will define the frequency of operation and the coupling of the waveguide modes. The distance between the slots will determine the overall radiation characteristics of the array, including the sidelobe level.
Slotted waveguide antennas have higher power handling capacity than patch array antennas. They also feature lower losses compared, which increases the overall gain and efficiency of the radar system. Another advantage is their inherent wide bandwidth, which makes them especially suited for large bandwidth, high resolution applications.
On the other hand, waveguide antennas can be more complex than other types of antennas, and they may require more space. The half-wavelength raster required for maximum field of view cannot be respected, which can lead to reduced angular resolution.
One of the main challenges of waveguide antennas is that they require careful design and manufacturing, as they are highly sensitive to small geometrical changes and fabrication tolerances. Accurate simulation models and passive antenna characterization will be capital for a successful design.
They also require an additional assembly process with high precision positioning over the board, as well as customized adapters to transfer the signals from the transceiver to the waveguide. Yet, with the advances of manufacturing technologies, it is now possible to fabricate slotted waveguide antennas with small size and flat form-factor, using relatively low-cost technologies, like metalized plastics.
Linear patch arrays and slotted waveguide arrays are probably the two most common types of antennas used in automotive radar applications, but they have different characteristics that make them better suited for different types of applications.
Ultimately, the choice of the type of antenna to be implemented is a radar system will depend on the specific requirements of the application, such as the required range and field of view, and the physical constraints for the integration of the radar module in the vehicle. Cost, manufacturability and system complexity will also be decisive factors.
Slotted waveguide arrays may be more suited for long-range and imaging radar systems requiring high power, gain and accuracy. On the other hand, patch array antennas may be preferred in shorter-range radar applications, for which small size and wide beamwidth are more important.
Ultimately, the choice between them depends on the specific requirements of the application, such as the required range and field of view, and the physical constraints for the integration of the radar module in the vehicle. Cost, manufacturability and system complexity will also be decisive factors.
Other options, such as dielectric and metallic lenses have also found their niche in the automotive market and may have a comeback if higher frequency bands are introduced. New applications like in-cabin side impact sensors with low range and cost may call for antennas in package.
Independently of the type of antenna that is chosen, there is still an issue to be solved: how to optimize the transfer of the radar signals from the radar transceiver to the antenna and vice-versa. Renesas is working with partners and stakeholders to reduce the losses in the RF interfaces of its radar MMIC products to improve the overall system performance. This will be the topic of the next blog entry.
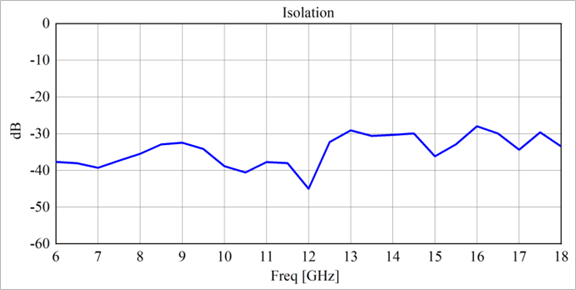
Corrugated Feed Horn Antenna Join thousands of engineers who never miss out on learning about the latest product technology.